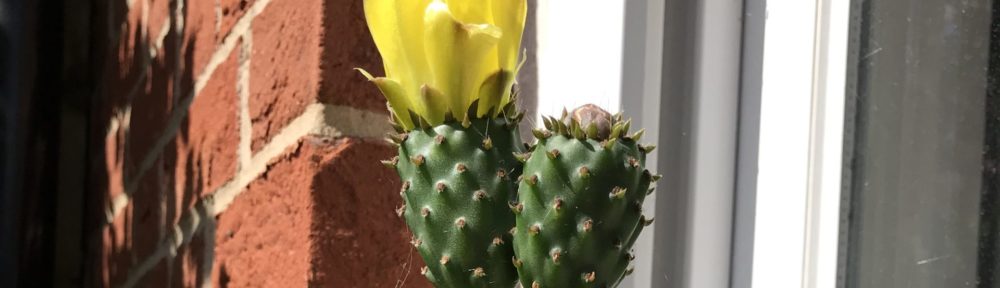
New Challenger for Sustainable Ethanol Production in Industrial BiorefineriesNew Challenger for Sustainable Ethanol Production in Industrial Biorefineries
To tackle climate change and achieve Sustainable Development Goals, integrating a circular economy with sustainable biomass use is vital. Recent studies highlight the efficiency of using nopales (prickly pear cactus)
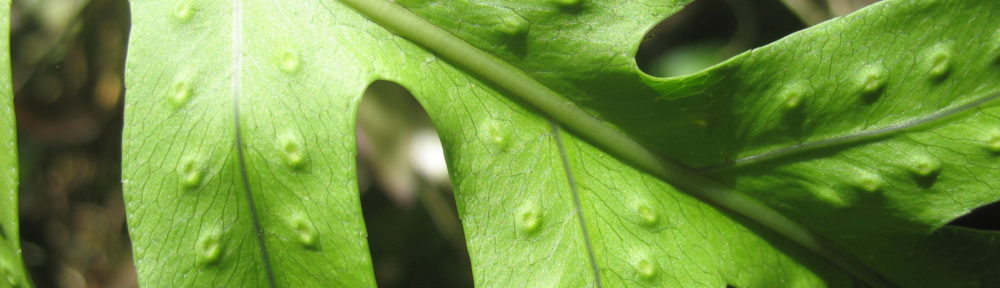
New process for commercial chemicals from biomassNew process for commercial chemicals from biomass
Taking an environmentally friendly idea from the lab and making it available to the public is not a straightforward matter. But hopes open, as shown by this novel process for adding
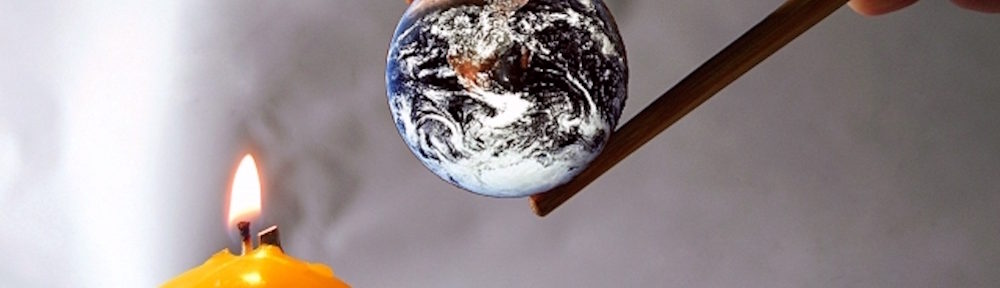
The planet is warming up… How can engineering help?The planet is warming up… How can engineering help?
Guest Speaker: Dr Ernesto Hernandez (UNMC) With special response about engineering and environmental challenges by Dr Amirhossein Malakahmad (UT Petronas) Inspired in the exponential achievements in modern history, Ernesto Hernandez
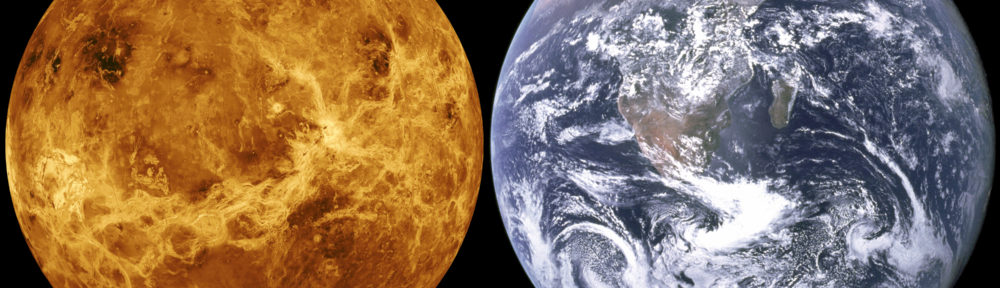
Saving the Earth from ending like Venus: on fire and raining sulphuric acidSaving the Earth from ending like Venus: on fire and raining sulphuric acid
We show a new way to save our planet from global warming. To tackle this problem, We focus on a natural approach to capture the smoky carbon dioxide released by industrial plants and make chalk out of
Biotechnological challenges in agriculture: addressing drawbacks, failures, and limitations.Biotechnological challenges in agriculture: addressing drawbacks, failures, and limitations.
Biotechnology has revolutionized agriculture with promises like increased yields and pest resistance but also brings drawbacks such as genetic monoculture, pesticide-resistant pests, and socio-economic disparities. These issues, alongside regulatory and
Challenges and Limitations of Vertical FarmingChallenges and Limitations of Vertical Farming
Vertical farming, a novel agricultural approach, faces challenges including high costs, dependence on artificial lighting, limited crop variety, pest issues, and systemic barriers, but remains a hopeful solution to food
Navigating the Drawbacks and Challenges of Bioenergy from MicroalgaeNavigating the Drawbacks and Challenges of Bioenergy from Microalgae
One of the primary drawbacks of bioenergy from microalgae is the scalability and cost-effectiveness of production. While microalgae can be cultivated in various environments, including freshwater, seawater, and wastewater, scaling
Job Research Internship BioRenewablesJob Research Internship BioRenewables
Role Global challenges require global actions to achieve Sustainable Goals while supporting a circular economy. Biomass is a renewable versatile resource that can be used to produce heat, power, transport